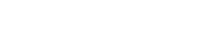
Will Earth Run Out of Oxygen
Season 13 Episode 4 | 16m 53sVideo has Closed Captions
The key enzyme behind photosynthesis isn’t actually all that great at its job.
Plants eat sunlight and air to make life. But the key enzyme behind it all, called RuBisCO, isn’t actually all that great at its job. Let’s talk about how photosynthesis really works, why oxygen isn’t coming from where you think, and whether we can fix the biggest flaw in one of Earth’s most essential processes.
Problems with Closed Captions? Closed Captioning Feedback
Problems with Closed Captions? Closed Captioning Feedback
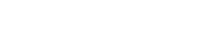
Will Earth Run Out of Oxygen
Season 13 Episode 4 | 16m 53sVideo has Closed Captions
Plants eat sunlight and air to make life. But the key enzyme behind it all, called RuBisCO, isn’t actually all that great at its job. Let’s talk about how photosynthesis really works, why oxygen isn’t coming from where you think, and whether we can fix the biggest flaw in one of Earth’s most essential processes.
Problems with Closed Captions? Closed Captioning Feedback
How to Watch Be Smart
Be Smart is available to stream on pbs.org and the free PBS App, available on iPhone, Apple TV, Android TV, Android smartphones, Amazon Fire TV, Amazon Fire Tablet, Roku, Samsung Smart TV, and Vizio.
Providing Support for PBS.org
Learn Moreabout PBS online sponsorship- [Instructor] It never stops being totally magical to me that plants literally eat light and air, somehow transforming gas and sunshine into living bodies.
They're not that good at it.
At least not as good as they could be.
Slacker.
And that's because of one tiny molecule, the most critical piece in the machinery of photosynthesis.
And that molecule is terrible at its job.
What's even weirder is that almost none of our oxygen is produced by anything doing photosynthesis here on Earth today.
Yeah, the oxygen you're breathing right now is probably tens if not hundreds of millions of years old.
So where does the oxygen we breathe actually come from?
What are all these plants actually doing then?
And can we make photosynthesis even better by fixing the most important enzyme on Earth?
(quirky music) To answer these really big questions, first we gotta understand how photosynthesis really works.
Now you probably remember from science class that it goes something like this.
- Hit it.
(upbeat music) - Plant absorbs sunlight, breathes in carbon dioxide, and takes up water.
The green stuff in a plant does some cool chemistry to make sugar and exhale oxygen.
And that's not wrong, except this equation leaves out so much cool chemistry, like more steps and cycles and molecules than we could name in one episode.
And there are several different kinds of photosynthesis.
And it's not just plants that do it.
And it's such a complex series of chemical reactions that we're still figuring out how it works.
Like, we only uncovered one of its key steps for the first time ever in 2021.
So yeah, let's shine some light on what's really happening in photosynthesis, so we can really absorb the process, and breathe some fresh new knowledge.
Okay, I kinda overdid it there on the metaphors.
Photosynthesis is actually two separate processes, what I like to call 'the sun part' and 'the food part'.
The sun part is basically a really fancy way of moving electrons around, and it all starts with light.
All organisms that do photosynthesis use some kind of pigment to absorb light.
These pigments have a molecular structure that allows them to absorb certain wavelengths of light so they can harvest that energy and do things with it.
Plants, for example, have the green stuff, chlorophyll, a pigment that absorbs these wavelengths, but not these, and that's why most of the plants that contain it look green to us.
The process starts inside a special light-harvesting organelle called the chloroplast.
When that chlorophyll absorbs electromagnetic radiation from the sun, here, it excites some electrons.
But, those high-energy electrons are unstable.
So they get passed down the line to other photosynthetic pigments, kind of like a game of electromagnetic hot potato.
But chlorophyll needs to replace the excited electrons it gave up.
To do that, the chloroplast takes water brought up from the roots, splits it apart, and steals some replacement electrons to give back to chlorophyll.
The leftover shards from that torn apart water are some protons or hydrogen ions, which the chloroplast stores for something important a little bit later, and this oxygen, which gets thrown away.
The electrons being hot potato-ed down the line are pumping more protons inside the chloroplast, concentrating them.
Until eventually, the electrons end up in this molecule, NADPH, basically a molecular battery that chloroplasts use to store up electrons charged by the sun.
As for all those protons that have been building up inside, they flow through this little molecular machine, like a water wheel, powering a factory that makes ATP, the energy currency that powers most cellular processes.
And this is the end of what I call the 'sun part' of photosynthesis.
So we've taken care of these parts of our familiar equation, but we still haven't done anything with this CO2, or figured out where this comes from, that yummy, yummy sugar the plant uses to power all its cellular processes.
So let's take a look at the other half of photosynthesis where the 'food' part begins.
- Yummy.
- Aka the light-independent reactions.
Because this is where we'll find the fatal flaw in photosynthesis.
We all know that plants absorb CO2 from the air around them.
They do that through little pores called stomata.
They're kinda like the pores on our skin, except stomata let water and gases like CO2 and oxygen in and out.
And that CO2 will become food for the plant, thanks to this.
An enzyme complex called rubisco, arguably the most important protein machine on Earth.
Now, rubisco's job is to pull the carbons off the CO2 coming in through the stomata.
It combines those carbons to make a kind of sugar, but it's not glucose, not quite yet.
That's why we need the ATP and NADPH from 'the sun part' of photosynthesis, they provide the energy and electron boost that transforms that intermediate sugar all the way into glucose, the sugar the plant needs to feed itself and build its body.
Now we've accounted for all the parts of our photosynthesis equation.
Done and done.
Harvesting electromagnetic radiation to energize electrons, rip apart water into subatomic ingredients, charge up molecular batteries, and use that energy to turn air into a living plant.
It's a truly remarkable process of biological chemistry, and at the heart of it is rubisco.
This whole process of a photosynthetic organism absorbing CO2 and turning it into solid stuff is called carbon fixation, taking carbon from the atmosphere and fixing it in place.
And rubisco is the bridge between those inorganic and organic worlds, taking nonliving carbon from the air and transforming it into carbon molecules that all life forms depend on to survive.
By some estimates, rubisco is the most abundant enzyme on Earth, and every year it fixes around 100 to 115 billion tons of carbon.
That's impressive.
- Oh yeah!
- Which is why it's so surprising that rubisco is actually not very good at its job.
Yep, rubisco is the slacker, the weak link of photosynthesis that I was talking about.
It's an enzyme, and enzymes are things that speed up chemical reactions without being consumed themselves.
So in photosynthesis, rubisco helps CO2 become other carbon-containing molecules that eventually become glucose.
Now, for an enzyme to be 'good' at its job, it needs to be both quick and picky or in scientific terms, 'highly selective'.
In other words, it's gotta be really good at grabbing on to the things it's supposed to, and nothing else.
And it's gotta be able to bind and release, bind and release, over and over at a fast rate to keep the reaction ticking along.
But rubisco doesn't do either of those things well.
For starters, it's incredibly slow.
It can only process between 3-10 molecules per second, which, I know, sounds fast until you consider that most enzymes can process thousands of molecules per second.
And rubisco also gets confused.
It's really bad at telling the difference between carbon dioxide, the thing it's supposed to bind to and ironically, oxygen.
You can think of rubisco as someone who, I don't know, thinks all guys with glasses who talk about nerdy stuff on YouTube are the same person.
Because we are not, okay?
We are not.
It's rude, stop.
When rubisco binds to oxygen instead of CO2, it actually ends up consuming energy instead of making the energy the plant needs.
And in some kinds of plants, rubisco gets it wrong more than 20% of the time.
Scientists think rubisco's inefficiencies are probably the reason it's so abundant, because plants need SO much of it to get anything done.
In fact, many plants have evolved workarounds because of rubisco's inefficiencies, and they do photosynthesis a little differently.
C3 and C4 plants package CO2 into molecules with 3 or 4 carbons before it gets to rubisco, and essentially putting that carbon on a silver platter saying, "Here it is, here's the right thing.
Just use this!"
And in CAM photosynthesis, like what cacti and succulents use, CO2 is stored as an acid before it's released for photosynthesis.
So how did we end up with a process that everything depends on, done by an enzyme that isn't very good at what it's supposed to do?
To understand that, we gotta go back to somewhere between 2.5 to 3.5 billion years ago when there was no oxygen in Earth's atmosphere whatsoever.
That is, until a tiny green hero came onto the scene.
Believe it or not, we have microbes to thank for our entire existence.
Earth's earliest life forms could do a primitive kind of photosynthesis that didn't produce oxygen.
It's called anoxygenic photosynthesis.
But somewhere along the line, ancient microorganisms evolved a new process, using an early form of rubisco, which let them turn light into fuel by using CO2.
This new process produced oxygen as a happy byproduct.
And this is the origin of rubisco's achilles' heel.
It can't tell the difference between O2 and CO2 super well because when it first evolved, it didn't have to.
There was so little oxygen in Earth's early atmosphere that there was no need to tell the two molecules apart.
And the mega-cool thing about bacteria being the first oxygen producers on the scene is that these single celled creatures are still our number one producers of oxygen.
People often say that trees are our planet's lungs, providing us with oxygen, but land plants only produce some of the oxygen given off by photosynthesis.
The rest, somewhere between 50 and 85% of the planet's oxygen comes from our oceans and the photosynthetic organisms that live in it.
Some of these are big plants and algae, but most are teeny tiny little dudes like this guy, prochlorococcus.
This single bacterial phytoplankton is both the smallest and most abundant photosynthetic organism on Earth, at least by number.
This one organism produces as much as 20% of the Earth's oxygen.
Not bad.
But all of this talk about which organisms make what fraction of Earth's oxygen, this whole conversation leaves out a hugely important part of the picture, photosynthetic organisms don't just produce oxygen, they consume a lot of it too.
Now see, when photosynthesis is done and the microbe or the tree has produced those sugars, it still has to use them.
Sure, it's made its own food, but just like you, I, a dog, or a frog would break our food down into energy, a photosynthesizer also needs to break its food down to actually release the energy and use it.
Just like us, plants have mitochondria and inside those mitochondria, plants consume oxygen to break down their food sugars into energy they can use for going about the business of being alive.
To do this, plants actually re-inhale about half of the oxygen they exhale.
The other half is most likely absorbed by other living things that live right around those photosynthesizers.
In the case of forests, that's other microbial life in the soil, and in the case of oceans, that's other marine life.
What this means is, in pretty much any biome on Earth, when you add up the oxygen produced and subtract the oxygen that's consumed, it comes out very close to net zero, at least on human timescales.
When I first heard this, I was shocked.
But it's true.
All this photosynthesis happening on Earth today, in the oceans and on land, almost all that oxygen gets used up by stuff that's not us.
And this is partly rubisco's fault.
When rubisco accidentally binds oxygen instead of CO2, instead of making energy, it actively consumes energy and releases CO2 back into the atmosphere.
It's like photosynthesis run backwards.
You had one job, and this is what you do?
If we could reduce the number of mistakes that rubisco makes, that'd mean plants growing better and pulling more CO2 from the atmosphere.
Those are both really exciting things.
Because as the world's population continues to grow, we need to feed more and more people.
Plus, I don't know if you've heard, we're facing a climate crisis because of the amount of carbon dioxide in our atmosphere.
So making rubisco better at its job could be a massive help in facing two of our world's biggest challenges.
Some scientists are actively working to engineer and improve rubisco for this purpose by carefully tweaking parts of the protein to work faster and be more selective.
And this work could eventually even lead to synthetic photosynthesis machines.
But could that also be bad?
Would a better rubisco lead to an increase in oxygen in the atmosphere because plants would be producing more oxygen than they're consuming?
Well, maybe.
But not in our lifetimes.
These days, Earth's atmosphere holds a comfortable 21% oxygen, and that number's been stable for pretty much the last 350 million years.
But if all the photosynthetic ecosystems producing oxygen today are basically using as much as they make, where did all this oxygen come from?
How did we get from tiny microscopic organisms first learning how to use rubisco to this atmosphere?
The answer?
Very, very slowly.
Because photosynthesizers do produce a tiny, miniscule fraction more oxygen than they consume.
So over literally hundreds of millions of years, the amount of oxygen slowly built up.
And throughout geologic time, Earth's oxygen levels have had some pretty big ups and downs, with lows at almost zero and highs at 35%, way higher than today.
But Earth has a pretty nifty oxygen balancing system to even out these spikes and dips.
Over long periods, oxygen can be absorbed and released by minerals in the Earth and the oceans.
But these changes are taking place over the course of hundreds of millions of years In fact, Earth's natural oxygen balancing system for oxygen is so powerful, some scientists estimate that if all organic matter on Earth were burned up in an instant, the Earth's oxygen concentration would only drop by less than 1%, and would stay stable for millions of years.
Of course, we'd have plenty of other problems, but a short-term lack of oxygen wouldn't be one of them.
The oxygen we breathe today was first exhaled out by bacteria billions of years ago, with more and more of it accumulating little by little, thanks to new organisms evolving the magic trick we call photosynthesis.
Everything alive today is simply recycling those first breaths of fresh air.
I don't know if any of this can ever really get across just how nuts it all truly is, using nothing but water, sunlight, and air, plants build their own bodies.
And those bodies are then something we and other consumers eat and break down for energy in our bodies, all using oxygen first exhaled by bacteria billions of years ago.
I mean, everything in the world eats something, and when you get down to the very bottom of the food chain, that something is light and air.
So even though photosynthesis has some room for improvement, it's a reminder that life isn't perfect.
It's just good enough.
And that's pretty great too.
Stay curious.
Support for PBS provided by: